When designing generator systems, electrical engineers must ensure that the generators and the building electrical systems that they support are appropriate for the specific application. Whether providing standby power for health care facilities or prime power for processing plants, engineers must make decisions regarding generator sizing, load types, whether generators should be paralleled, fuel storage, switching scenarios, and many other criteria.
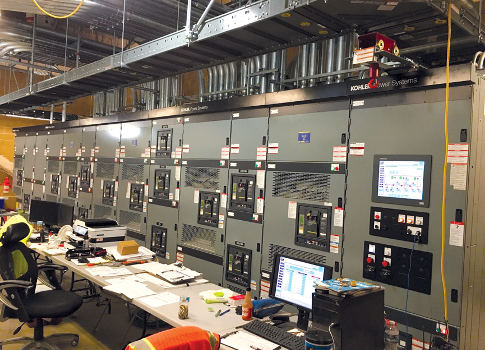
Learning objectives
- Learn best practices for paralleling generators, touching on dependability, cost savings, efficiency, synchronization, and other aspects.
- Know the requirements for emergency, standby, and backup power loads.
- Explain the benefits of parallel power-generation systems.
Editor’s note: Because of the extent of this topic, this article is divided into three parts:
Part 1: The need for backup power, code requirements, why diesel is preferred, generator ratings, and the benefit of paralleling generator systems.
Part 2: Paralleling switchgear, their components, and common paralleling modes.
Part 3: Installation considerations, interconnection with the utility, and generator sizing. Also, two existing parallel generator systems will be presented and their paralleling elements highlighted.
As defined in Part 1, “Paralleling generator systems,” in the December 2016 issue of Pure Power, paralleling is the operation in which multiple power sources, usually two or more generators, are synchronized and then connected to a common bus. Paralleling switchgear (PSG) is a combination of protection, metering, control, and switching elements acting as an integrated system to control the distribution of power for the following systems:
- Emergency systems
- Legally required standby systems
- Critical operations power systems (COPS)
- Business-critical systems, also referred to as optional standby systems.
PSG can be deployed at various voltages. The voltage selected is a function of system capacity, standard voltages for the region, and utility voltages. The systems can be of switchboard construction, metal-enclosed construction, or metal-clad construction. The applicable standards depend on the voltage and type of construction.
For low-voltage PSG:
- UL 891-2005: Switchboards for dead-front switchboards
- UL 1558-2016: Standard for Metal-Enclosed Low-Voltage Power Circuit Breaker Switchgear and ANSI/IEEE C37.20.1 for metal-enclosed low-voltage power circuit breaker switchgear.
For medium-voltage PSG:
- ANSI/IEEE C37.20.2-1999: IEEE Standard for Metal-Clad Switchgear for metal-clad and station-type cubicle switchgear.
- The equivalent Canadian standard for all the above is CSA C22.2 No. 31-2014: Switchgear assemblies.
PSG components
PSG consists of breaker compartments and control compartments (see Figure 1). These can be integrated into one lineup or installed in separate enclosures. The latter is preferred to lower the arc flash hazard risk category. System operators spend most of their time near control compartments; therefore, the recommended approach is to locate the controls compartments outside the arc flash boundary of the breaker compartments.
Paralleling system breakers: Regardless of the layout of the switchgear, the following are used to label basic breaker compartment requirements for parallel switchgear application:
- Utility main breakers—breakers directly fed from the utility source
- Generator breakers—breakers directly fed from the generator source
- Generator main breaker—breakers that tie the generator bus to the load bus and are used for transfer between the two sources (generator source or utility source)
- Load feeder/distribution breakers—breakers that directly feed loads or transfer switches
- Tie breakers—breakers between similar source breakers. These could be the load-bus tie or generator-bus tie breakers.
Protection: The protection requirements for PSG depend on the size of the generators, system voltage, and the required level of system reliability. Low-voltage circuit breakers with built-in trip units provide the protection necessary for low-voltage feeder circuits. Utility and generator circuits require a more sophisticated protection scheme than low-voltage circuit breakers with integral trip units can provide; therefore, breakers with separate multifunction protective relays are provided. For medium-voltage breakers, relaying systems normally are employed to provide the necessary protective measures for all breakers (see Figure 2).
These protections include:
- Generator protection. Generators normally require the following protective functions as indicated by ANSI device numbers 25, 27, 32, 40, 46, 49, 50P, 50/51G, 51V, 59, 67G, 81O/U, 87G, and BF (breaker failure). (See Table “ANSI device numbers/description.”)
- Generator main and generator bus tie breaker protection. Utility circuits normally require the protective functions provided by the device numbers 25, 27, 32, 50/51P, 50/51G, 59, 81O/U, and BF.
- Incoming utility protection. Utility circuits normally require the protective functions provided by the device numbers 25, 27, 32, 50/51P, 50/51G, 59, 67, 81O/U, and BF.
- Feeder and load bus tie breaker protection. For feeder circuits in medium-voltage systems, minimum requirements are 50/51P, 50/51G, and BF.
- Failure to trip. Backup protection must be provided for the case where a breaker fails to operate when required to trip (breaker failure). This protection consists of a current detector in conjunction with a timer initiated by any of the protective relays in the generator zone. If the detector shows that the breaker has not opened by the time the specified time delay has passed, the breaker failure relay will initiate, which trips the backup breakers.
Control compartments:
- PSG control compartments include:
- Master/system control compartment—generator optimization, load add/shed, system status annunciation, user human-machine interface (HMI), and transfer control are located here to control the paralleling of generators.
- Generator control compartment—used for generator starting and stopping, voltage control, and to provide annunciation of speed, output, engine and generator status, alarms, and shutdowns. One control compartment is required per generator.
- Utility control compartment—used for loading control between the utility source and the generator source, power management, and providing transfer device status. One utility control compartment is required per utility source.
- Mimic panel—used to display system one-line status when using an operator interface panel. This function is incorporated in the control system, which is normally a 15- to 19-in. color touchscreen HMI.
Load management: Load management, also called add/shed, is controlled by the PSG’s master controller and is very important for the efficient operation of the engine-generator. Loads are added by preassigned priorities based on the available online capacity, thereby maximizing the noncritical loads supported by the generator system. Load/add also removes noncritical loads when the capacity of the power supply is reduced or unable to support the entire load. In addition, if no actions are taken when a generator fails the generator bus experience (a severe underfrequency or undervoltage situation due to an overload condition), there will be a complete power loss due to an underfrequency or undervoltage shutdown. The load-management system will remove the noncritical loads quickly and in an orderly fashion, thereby allowing the bus frequency and voltage to recover within a few seconds, enabling the critical loads to remain on standby power.
Certain metered values are required for load management: watts, watt demand, watt-hours, and engine run time. The PSG requires power meters to provide these metered values via analog signal and/or communication. For precise load management, individual power meters are needed to provide accurate real-time metered values. Whenever there is a loss of voltage and changes to frequency, these values must be captured and used by the master controller to transfer/retransfer, add, or shed loads. Engine run time can be used to distribute usage of all generators evenly. Generators can be sequentially or randomly loaded and unloaded based on this information.
Engine control: There are two major engine/generator controls required by the PSG for proper functioning:
- Engine governor controller—controls the speed and output of the engine. Most major engine manufacturers have their own matched engine governor controller and prefer to mount it near the engine in the control cabinet. In these cases, the programmable logic controller (PLC) in the PSG would only provide the enable signal; the engine governor controller would control the engine-generator.
- Voltage regulator—controls the voltage and the reactive power of the generator. Voltage regulators are most often mounted near the generator in the terminal box. There is a need to have an automatic synchronizer to send control signals from the PSG to the voltage regulator and engine governor controller to bring the generator into synchronization with the generator bus. The synchronizer normally is mounted in the PSG and is shared between generators. Synchronizing switches are used to connect the synchronizing equipment to the correct line and load bus.
Master control: PLCs are the heart of the PSG. To ensure reliability, the PSG will have one controller per generator and a redundant or hot backup PLC system for the master controller. Protective relays, metering, control, and display devices are connected to the system, and the PLCs are daisy-chained to each other. The master PLC controls the transfer switches or breakers for load add/shed. The generator PLCs communicate with the engine-generator’s controllers via specific gateways. Alternatively, complete manual control could be employed as backup for the PLC. Critical start/stop, alarm, and shutdown signals can be hardwired to meet the requirements of NFPA 110-2016: Standard for Emergency and Standby Power Systems. During master PLC failure, the generator PLC will provide the generator start signals, automatically synchronize all generators, and connect the first-priority loads. The lower-priority loads will have to be connected manually.
Common paralleling modes
Common switchgear paralleling modes include island, manual, system testing, no-load testing, and on-load testing.
Island mode (isolated from the utility). After utility power is lost, the PSG will disconnect from the utility. The PSG contains relays that monitor the change in frequency and/or voltage of each utility connection. If the relays detect a change in either frequency or voltage outside of a set threshold, indicating that the utility sources are collapsing, the relays will trip the utility’s incoming circuit breakers that connect the backup paralleling plant with the rest of the utility network. When the utility is totally disconnected from the PSG, the generators will be started. A typical sequence of operation for standby systems where the generators serve as backup to the main (utility) source is outlined below.
The first generator to reach rated voltage and frequency is connected to a dead bus without having to wait to synchronize. This generator must be sized to provide power to the emergency and life safety loads. For emergency systems, this generator must supply the bus within 10 seconds.
As the remaining generators reach proper speed and voltage, they are paralleled to the generator bus. The remaining generator breakers are then closed onto the generator bus—typically, within 20 to 60 seconds.
The PSG generator control system controls the addition of nonemergency loads to the PSG load bus based on available generator capacity and the assigned priority of the block loads. As each additional generator parallels to the bus and the PSG detects that there is sufficient generator capacity, the PSG closes the breaker for Priority 2 loads, then Priority 3 loads, Priority 4, and so on. This will continue until all generators and all loads, within the limits of capacity, are online.
When the generators are synchronized, the PSG load-sharing module will control the generators. The load-sharing modules are connected to each generator and automatically adjust the speed (frequency) and voltage so that each generator shares the load evenly, keeping the desired frequency and voltage within acceptable limits. Most load-sharing systems today are isochronous, meaning that the voltage and frequency are held constant.
In closed transition back to the utility, the PSG will parallel the generators with the utility. The frequency, voltage, phase angle, and phase rotation of the generator system must match the utility within prescribed limits of the governing utility before the generator system can be paralleled. The transition time for closed transition depends on the governing utility. Most require a quick make-before-break closed-transition time with 500 milliseconds maximum transition time. Some utilities require a soft transfer of load with a maximum transition time of 2 to 3 seconds.
Manual mode. A key consideration in assessing the reliability of a system is the ability to operate the system when certain components have failed. Having a predefined sequence of operations for an operator to follow in a worst-case scenario to manually provide power to loads is often a requirement in paralleling applications. An operator should be able to manually start generators, initiate synchronization, and close paralleling breakers. Manual operation does not mean that the generator control is not operating. It means only that functions are user-initiated rather than system-initiated. All system-protection functions will still be active. The control will not allow a paralleling breaker to close if the generator and the paralleling bus are not in phase with each other. Furthermore, the control will not allow the exporting of generator power to the utility.
System testing modes. System testing is a very important requirement for emergency/standby systems. NFPA 110 emergency generator testing requirements set safety standards to protect commercial building occupants by making sure generator-powered backup lighting will operate as expected. Monthly and yearly tests are performed on generator systems whose failure could result in death or injury. Should a generator system fail this monthly test, it must be repaired immediately and re-tested until the emergency system passes.
No-load testing. This type of testing is performed with the generators started, synchronized, and connected to the generator bus without taking on load. This test will not have any effect on the load fed from the utility and can be scheduled to occur at regular intervals (engine exercise setting). No-load testing determines whether the engine can start when given a signal to do so, but will not indicate whether the generator will actually carry the load. An on-load test can determine if the generator system can carry the critical load reliably.
On-load testing. This type of testing is performed where the load is gradually transferred from the utility to the generators via closed transition, run for a period of time, and then transferred back to the utility. Running a generator system under actual facility load is the only true way to test its capability to operate as specified. There are many engine and generator components that must see real operating conditions to provide confirmation of their ready state.
Parallel with utility
The PSG can parallel with the utility to perform peak shaving. However, it is rarely accomplished because this will require a utility agreement. Peak shaving is done to avoid the high cost of peak demand charges. Upgrading the generator units to prime rating and increasing the fuel system’s size for the longer run times would be necessary. Also, running the generators more than 300 hours/year can invoke higher environmental and acoustical requirements.
In peak-shaving mode, the generators supply all the power demand after the demand exceeds the peak-demand threshold. If the generating capacity is less than the load demand, the generators are run at full load and the balance demand is supplied by the utility.
Generator integrated-control systems
There are manufacturers that provide generator integrated-control systems where the generators have plug-and-play technology. This allows the generator controller to be connected together with just control cables to a generator power-management controller. The power-management controller is used to interface with each generator in the system as well as the downstream automatic transfer switches (ATSs). After the control cables are interconnected, the user connects the generator output power cables to standard 480 V low-voltage switchgear to make a common generator bus. The integrated-control system is a factory-designed, tightly controlled and vetted system with strict parameters to set. There is very little or no customization of the software allowed. The integrated generator controllers have:
- A speed governor
- A voltage regulator
- An engine controller
- Automatic synchronization of the generators
- Load sharing (kW and kVAR)
- Onboard generator and generator-feeder protection
- PC-based operation screens
- Control of individual generators
- Priority load shedding
- The ability to provide an N+1 generator redundancy
- The capacity to easily add future generators
- Lower cost than traditional paralleling systems.
This type of installation typically includes a simple generator standby system that can only perform open-transition transfers through the downstream ATSs. The opened-transition ATSs provide the necessary utility-interface protection. As a consequence, there will be an interruption of power going from generator power to utility. Load-bank testing also will be required to test the generator system at least once a year per NFPA 110. Integrated-control systems are not as robust as the standard PSG, which is programmed with numerous failure-mode schemes, such as breaker failures.
Leslie Fernandez is senior project engineer, electrical at JBA Consulting Engineers. He has more than 30 years of engineering and design and field experience including medium-voltage distribution systems for military, mining, tunneling, food manufacturing, power-production facilities, high-rise facilities, and casino-resort complexes.