High-performance building design in health care facilities may teach engineers how to design other building types
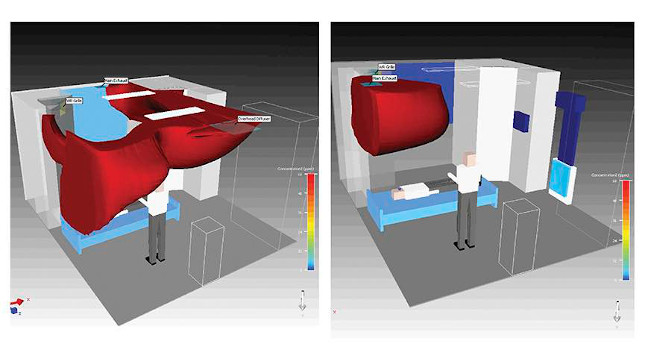
Learning Objectives
- Understand the strategies used in health care to control airborne infectious aerosols.
- Learn how high-performance hospitals meet prescriptive code requirements for infectious control, while still satisfying high-performance building energy targets.
- Review opportunities for applying high-performance health care strategies to other building typologies to increase their performance based on changing criteria for successful projects.
High-performance buildings have always been intricate, complex projects that require attention to detail, collaboration and the management of competing interests. Now, enter a new layer of design requirements for incorporating pandemic control measures and pandemic resiliency into buildings.
In health care, mechanical building system design is largely governed by codes and standards. In the United States, guidelines from ANSI/ASHRAE/ASHE Standard 170: Ventilation for Health Care Facilities are in effect for air system requirements. In Canada, CSA Standard Z317.2: Special requirements for heating, ventilation and air conditioning systems in health care facilities plays a similar role.
These documents enforce specific minimum requirements for the control of airborne infectious disease that underscore an overarching design philosophy that promotes infection control and prevention. In the design of hospitals, the integrated design team has always looked for ways to meet the prescriptive infection control requirements while still creating sustainable and energy efficient high-performance buildings.
By leveraging highly evolved health care design solutions, engineers can help jump the curve and bring energy-efficient, sustainable and pandemic-control design measures to high-performance buildings in all sectors.
High-performance building infectious disease design
One of the key concerns in health care is with the aerosolization of an infectious disease. Imagine someone coughs, sneezes or even just talks, spreading an infectious disease that can be transmitted from the carrier via large or small droplets. If that droplet evaporates before contacting a surface, the nuclei can be aerosolized and the pathogen becomes airborne, potentially riding air currents for hours before settling on a surface or entering a host.
In the typical acute care health care environment, approximately 10% of all inpatient rooms are designed as airborne infection isolation rooms — rooms designed for the isolation of patients infected with organisms spread by airborne droplet nuclei less than 5 micrometers.
In the United States, ASHRAE 170 requires that for airborne isolation rooms have “exhaust grilles in the patient rooms located directly above the patient bed” (clause 7.2.1.d).
Similarly in Canada, CSA Z317.2 requires “directional airflow within the room such that clean supply air flows first to parts of the room where workers or visitors are and then flows across the infection source (i.e., patient area) to the exhaust” (clause 6.10.4.2.1).
The evidence and rationale behind the prescriptive requirement for this directional airflow is well documented and the use of these measures is commonplace in specific health care settings, designed to contain highly contagious patients.
Enter the world of pandemic planning and this rationale is being further extended to nonisolation rooms in both health care and nonhealth care buildings. The ASHRAE Position Document on Infectious Aerosols issued April 14, 2020, in response to the COVID-19 pandemic cites multiple research papers in its documentation of the fact that “directional airflow can create clean to dirty flow patterns and move infectious aerosols to be captured or exhausted.”
The document goes on to conclude that various strategies, including directional airflow, have been found effective at controlling transmission and states explicitly that “design engineers can make an essential contribution to reducing infections aerosol transmission through the application of these strategies.”
What options do building designers have to provide directional airflow? There are many, but first it is important to distinguish the difference between 100% outside air and recirculating air systems.
Building ventilation systems
Many building ventilation systems use distributed fan coils or heat pumps. These systems introduce a small percentage of outside air as required to satisfy space ventilation and make up air requirements, with the majority of air being locally filtered, tempered and recirculated in the space. The efficacy of the local filtration depends on the minimum efficiency reporting value, or MERV rating of the filter.
Typical distributed fan coils or heat pumps are provided with a maximum filter rating of MERV 8. Current research is showing however that a minimum MERV 13 rating is required to provide any effectiveness for the control of infectious aerosols and that a high-efficiency particulate air-rated filters are the gold standard for control, with 99.97% effectiveness with particles as small as 3 micrometers. In fact according to the NASA study NASA/TM—2016–218224 Submicron and Nanoparticulate Matter Removal by HEPA-Rated Media°Filters, due to the diffusion, interception and impaction mechanisms, “HEPA filters are nearly 100% efficient at capturing the spectrum of particles down to the very smallest airborne particles.” However, due to physical size and pressure drops, standard distributed fan coils and heat pumps may not be able to accept this level of filtration.
With a standard recirculating air system — even if you have directional airflow from clean to dirty areas — mechanical engineers may not achieve the intended outcome, as a large portion of this now dirty air will be recirculated and potentially insufficiently filtered to protect against the spread of infectious aerosols.
Where building ventilation systems use central air distribution strategies, there may be an opportunity to operate these systems as 100% outside air supply and 100% exhaust without any recirculated air. Examples of such systems are variable air volume with reheat, dual duct or systems with decoupled thermal and ventilation strategies. In these cases, leveraging directional airflow can provide effective control of infectious aerosols, however, this may result in a significant energy penalty associated with overventilation.
But how do you actually achieve the directional airflow? In standard overhead mixing systems, the fundamental basis for how these systems work is based on fully mixing the air in the occupied spaces. As such, achieving effective directional airflow may not be practical.
Displacement ventilation in health care
There is one ventilation system in particular that has been tried and tested in the health care setting as an effective means of providing directional airflow and superior energy performance.
Traditional overhead mixing systems both supply air from the ceiling and exhaust air from the ceiling level. The air from the ceiling supply diffuser must be discharged with relatively high velocity to reach the occupied zones and not simply short circuit back to the exhaust grille. Air should be thrown at the rate of 400 to 800 feet per minute in the neck of the diffuser, slowing down to at least 150 fpm as the air starts to enter the occupied zone.
As this relatively high-velocity air is discharged from the diffuser, it entrains room air and creates, by design, a mixed air condition that results in a homogeneous distribution of supply air and room air and a fairly constant temperature over the entire volume of the occupied space. Due to this high level of mixing, the supply air from the diffuser can be provided at a relatively cool temperature (often as low as 55°F), without causing significant thermal comfort issues from occupants, as the in-room mixing helps to dilute the temperature.
In contrast, the concept of displacement ventilation is based on introducing cool (as opposed to cold) air into the space at a low level and a low velocity. In operation, it is common to see a minimum temperature bound of 63°F for the supply air and a maximum discharge velocity of 40 fpm. This cool air then pools at the lower levels of the occupied space and raises via buoyancy as it encounters heat sources such as occupants and equipment.
As the supply air warms and rises, it provides useful cooling in the occupied zone and helps to draw exhaled air up to the ceiling level, where the air is ultimately captured by the exhaust air system. This creates a stratification of air in which the occupied zone (the lower 7 feet of the room) is maintained within 3°F to 5°F of the average setpoint temperature for the space and the upper, unoccupied volume of the room is allowed to exceed setpoint temperatures.
Among other factors, the amount of supply air required in both overhead mixing and displacement ventilation systems is dependent on the required cooling loads.
For overhead mixing systems, the air system must be designed to condition 100% of the space cooling load, which is the sum of internal gains from occupants, lights, equipment, transmitted solar radiation and heat conduction through the room envelope.
For displacement ventilation, as the air is supplied from a low level and then stratified, at the room level the designer only needs to satisfy the space cooling load for the portion of heat gains within the occupied zone. As per the findings of ASHRAE Research Project-949, it was determined the effective cooling load in a space served by displacement ventilation could be de-rated. Specifically, the effective space load from occupants, desk lamps and equipment were only 29.5% of the total heat emitted from these sources.
The effective space cooling load from overhead lighting was only 13.2% of the total heat emitted from the overhead lighting — and the effective space cooling load from transmitted solar radiation and heat conduction through the room envelope was only 18.5% of the total transmitted envelope load. The key here being that this heat still enters the space, but as it stratifies and collects at the unoccupied high level, it does not need to factor into the room load required to be conditioned for human comfort.
The result of the deration in total space cooling load with displacement ventilation is the potential reduction in total air volume required to be supplied to a space. This reduction is somewhat offset by the fact that the air cannot be supplied as cold as it could be in overhead mixing systems, but most often the net effect is still a reduction in total cooling air volumes, duct sizes, fan sizes and fan power and chiller energy consumption.
In particular, the potential chiller energy savings cannot be understated. With a lower end supply air design temperature of 63°F in displacement ventilation, as opposed to 55°F with overhead mixing systems, in regions with favorable outdoor air conditions and low dehumidification demands, the system is able to operate in free cooling mode for more time of the year.
Further, when chillers are required to operate to provide cool air, they do not have to work as hard as warmer chilled water temperatures can be used. It is also noted that the as lower total air volumes are required, the cooling load airflow rate is often equal to or lower than the minimum ventilation rate airflow required. As such, these systems can also be efficiently configured to operate in 100% outdoor air mode. Were dehumidification demands drive cooling loads, face and bypass type cooling coil configurations can be used to improve system effectiveness.
High-performance building ventilation
There are significant potential capital cost and energy cost savings available from the deployment of displacement ventilation. This has been well established in the high-performance building design industry and many buildings are designed with displacement ventilation systems.
There is a further potential benefit of displacement ventilation, which is particularly relevant as it relates to post-pandemic design and is the virtue of directional airflow and 100% outside air.
In health care, much research has gone into modelling and understanding airborne aerosol transport mechanisms in the inpatient rooms and how the infection control aspects of displacement ventilation interplay with operating cost, first cost, environmental comfort and ventilation effectiveness. Most notably, the formal research performed under and referenced as part of the Stantec/M+NLB study entitled Health Care Ventilation Research Collaborative: Displacement Ventilation Research Phase II Summary Report was a game changer.
As part of this study, the multidisciplinary research team performed empirical (in-situ) testing with mock-ups, numerical modeling, validation and parametric numerical testing of displacement ventilation and traditional overhead mixing systems. They evaluated the impact of hot summer and cold winter conditions, the impact of air diffuser and grille locations, the impact of space ceiling height, the impact of internal occupant movement within the space and the impact of coughing within the space.
There were many key findings that resulted from this study, with one of the overarching findings that when properly designed displacement ventilation systems operating at four air changes per hour resulted in lower concentrations of contaminates in both the whole room and at the caregiver height, when compared to overhead mixing systems operating at six air changes per hour.
There are details associated with displacement ventilation systems that do need to be incorporated in design for proper operation. For example, if you have low ceiling heights, it will be challenging to achieve the design stratification. There is a practical limitation on how much cooling the system can deliver (estimated at 38 Btu/hour/square foot) if you have high solar gains that can create a hot spot on the floor that may short circuit the airflow. If you have envelope heat losses, these need to be satisfied with a decoupled system, as displacement ventilation does not provide heating.
However, these design details and other nuances associated with displacement ventilation can be addressed and, when correctly implemented, the result is a high-performance building mechanical system that saves capital cost, operational cost, provides superior indoor air quality and controls the spread of airborne infectious aerosols through the use of directional airflow.
Impacts to high-performance buildings
The concept and application of displacement ventilation in high-performance buildings is not new. Many buildings of all typologies have been successfully designed and built with various versions of displacement ventilation. In fact, references to displacement ventilation trace as far back as 1899 when the book Natural and Artificial Methods of Ventilation was first published and the concept of displacement ventilation was presented for use in schoolrooms. Even modern-day high-performance evaluation systems, such as U.S. Green Building Council LEED and WELL Building Institute WELL certification, place high value on the use of ventilation systems that provide superior indoor air quality.
The consideration now is will the pandemic resiliency of the building and the building performance in the control of potentially infectious aerosols start to factor into the evaluation of high-performance buildings? Along with ongoing conversations around energy consumption, greenhouse gas emissions, daylighting, biophilic design and climate resiliency, if high-performance building designers are looking to provide pandemic resiliency into their buildings, then we may see greater adoption and use of displacement ventilation in all of our buildings.