By collaborating with an interdisciplinary team, engineers can offer high-value, holistic solutions that promote resilient design for buildings and sites of all types
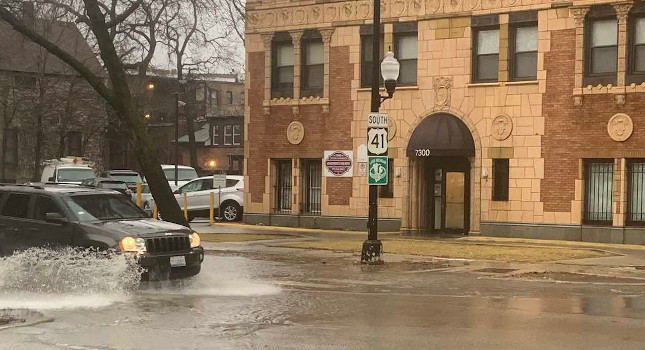
Learning Objectives
- Apply an interdisciplinary team of engineers and designers to identify shocks and stresses to design for resilience.
- Identify strategies for resilient design and evaluate strategy costs and benefits.
- Understand how MEP engineering solutions can offer resiliency for sites and buildings in the face of weather events.
Through resilient design, engineers can prepare for catastrophic events, address underlying stresses and adapt to changing conditions. This process of resilient design addresses physical infrastructure and organizational networks to adapt and rebound from the impacts of climate change and man-made disruptive events.
We believe that an integrated approach where mechanical, electrical and plumbing teams work collaboratively with architects, structural engineers, civil engineers and landscape architects is critical for effective resilient design. What good is a state-of-the-art facility if the power fails? A hospital that remains operational through a hurricane is only functional if it can be accessed via clear roadways.
Through frequent, collaborative problem-solving, a design team and building owners/operators can optimize solutions against potential threats, creating facilities and communities that are better poised to address the changing world around them.
Identify the threats
To appropriately plan and design for resilience, it is important to understand the risks and underlying vulnerabilities our communities are facing. The terms “shocks” and “stresses” classify hazards that impact buildings and communities. While commonly identified as natural disasters, shocks can also be a result of human behavior. Shocks can be catastrophic at local, regional and global scales. The enormity of their widespread devastation grabs our attention. It is important not to lose sight of the communities and people impacted during these events as the facilities and systems we design can help change their outcomes for the better.
Stresses are chronic, long-term issues and can be just as impactful as shocks. As stated by the Rockefeller Foundation, pioneer of 100 Resilient Cities, “Stresses are slow-moving disasters that weaken the fabric of a city. They include high unemployment, overtaxed or inefficient public transportation systems, endemic violence and chronic food and water shortages.”
Whether a strain on social structure, buildings or the natural environment, stresses heighten a community’s vulnerabilities and reduce its ability to respond to and recover from a disaster. We are experiencing the amplifying power of stresses during COVID-19. Evidence is clear that historically underserved neighborhoods with less access to health care, healthy foods and essential services are having disproportionately higher numbers of cases and death rates.
Stresses undermine communities and act as “threat multipliers” when they coincide with a shock, exacerbating potential devastation. For instance, a city’s ill-maintained drainage infrastructure reduces its capacity to handle severe storm events, increasing flood risk within the service area. With the interconnectivity of our natural and built environment, an initial hazard event can trigger subsequent or secondary hazards. With increased flood risk from a lack of maintenance, a severe storm can result in community flash flooding as the secondary hazard.
From there, ongoing hazards can occur as cascading events such as the floodwaters creating a landslide or a power outage. These cascading effects increase a community’s vulnerability and exposure to larger threats, compounding the challenges a community faces during recovery efforts.
Establish resilient design goals
At the beginning of every project, the interdisciplinary design team should work with the client to identify their goals for resilience. The project location will determine potential threats. Clients’ needs for operations during a disaster or during recovery often vary. A hospital and an office building will have different requirements for operations during a disaster.
This need for continuity of operations and ability to rebound from a disruption is called a “level of resilience.” Bringing all the design disciplines, the owner or client and the end users together in a workshop is the best way to understand the potential hazards, cascades and threats to life safety and facility operations.
Preparing for the workshop begins with identifying the possible shock events and underlying stresses that may impact the site, the building and its operations. Several resources for identifying threats by locale are listed at the end of this article. With these resources, the design team should develop a matrix of potential hazardous events. This matrix should include information on duration, severity, probability of occurrence and any secondary or cascading hazards. Completing this matrix with an interdisciplinary team will help all disciplines to think beyond a singular perspective.
After developing a baseline understanding of stresses and threats, the design team and client should think about how threats would impact the client’s operations and response. Role-playing the identified hazard events can be an effective technique. In many cases, a narrative structure that begins with defining normal operations and then walking through the immediate and long-term problems posed by each event is useful to compile a list of effects and possible solutions.
Consider studying groups of related hazards — such as short-duration weather events or events that would disrupt building power — to focus the discussion and draw out common solutions. Solutions may be a physical change in the facility design or organization or a change in operations.
As these discussions generate scenarios and possible solutions, keep in mind that the goal is not to fully develop a set of formal strategies, but to gather ideas and to gain an understanding of the client’s values and resilience goals that will lead to documented strategies.
Evaluate resilient design strategies
After playing out various scenarios, the design team can develop a threat assessment matrix to include possible responses and their associated costs. The matrix organizes potential strategies in a way that can reveal commonalities in solutions. This tool also provides the design team and the client with a method to assess the relationship between cost and probability of occurrence and can guide the client’s decision-making.
During this process, developing a menu of alternative solutions will help the client evaluate cost for the desired outcomes. Resilient design should not be approached as an “all-or-nothing” binary response. Rather, the menu of strategies can provide a range from simple, low-cost solutions to address a single aspect of disruption to complex solutions that bear a greater upfront cost, and ensure higher levels of operation throughout a hazard event. In between these two ends, the design team may find many different solutions that can be layered together to build a stronger, more resilient facility.
A big-picture approach to designing resilient MEP systems is essential. Collaborate with architects and planners, civil engineers and landscape architects, structural engineers and fellow MEP and fire protection engineers to develop the best methods to achieve resilient design goals.
Abiding by the proverb: “a chain is no stronger than its weakest link” (attributed to Thomas Reed’s “Essays on the Intellectual Power of Man” from 1768), redundant mechanical equipment is useless if the structure collapses or the mechanical room is under water. Bringing all the disciplines together results in the best solution.
Location of engineered systems
The location of major MEP equipment that is part of a resilient system is the most important factor because it must be able to function in the face of local threats. Coordinate the location of major equipment early in the new building design process so that the architect can allocate space and integrate into the aesthetic vision from the beginning. Here are some considerations for locating equipment:
- In regions prone to flooding, locate equipment above grade to prevent damage. For example, use pole-mounted electrical transformers.
- In regions prone to tornadoes or hurricanes, locate equipment inside to avoid the vulnerability of equipment and to prevent equipment from becoming projectiles. For example, locate cooling towers inside the building and duct their intake and discharge to the outside. Another example is to locate emergency generators in a hardened location inside the building and locate the fueling system in an area that can be easily accessed during a hazard event.
- Secure outdoor equipment to prevent damage from earthquakes, high winds or theft.
For resilient design of MEP systems, the design team should identify the most critical systems for operations and any additional systems supporting the most critical ones. These are a few example considerations for designing reliable MEP systems with redundancy:
- Heating is often more critical than cooling; however, cooling data closets is a highly critical function.
- Local redundant systems serving different areas may better enable critical operations versus a central utility plant that could be incapacitated.
- Power can be fed from multiple utility substations, so that if one goes down the building can still function.
- Backup generators can be powered by diesel fuel in lieu of natural gas in case gas service is interrupted.
- Power can be provided by off-grid solutions such as solar panels with battery storage if permitted by the authority having jurisdiction.
- Multiple incoming water services from different water mains can provide redundancy if one of the pipe mains fails or is shut down for repairs.
- Sanitary and storm drains can have multiple connections to different underground mains or on-site stormwater provisions can be made.
- On-site potable water and waste storage can be provided so that the building can fully function for a limited time without connection to utility piping.
Devising strategies for resilient design entails understanding the requirements of the owner and working with the local contractors and equipment manufacturers to understand system and equipment capabilities. Questions to ask for designing redundant systems include:
- What are the critical systems requiring redundant backups?
- What systems are necessary for others to function? For example, redundant boilers cannot function without power, therefore redundant power systems are required.
- How much redundancy is suitable for the project? Is an N+1 design acceptable so that if one piece of equipment is out of service, the building can still function?
- Do all systems need to function at 100% in a design hazard event? Can the redundant systems provide less function that maintains an acceptable level of operation?
Passive resilient design
Passive design that minimizes or eliminates reliance on power or mechanical systems is highly resilient. Examples of passive design strategies for enhanced resilience include:
- Use of natural site features and low-impact site design to drain water by gravity, thereby eliminating pumps, backup pumps and power requirements.
- High-performance building envelope to minimize heating and cooling requirements, reducing the need for redundant systems and emergency power.
- Operable windows to allow spaces to be habitable without air conditioning.
- Ceiling fans to provide comfort with minimal power requirements.
- High thermal mass building to reduce peak loads during cooling season and moderate indoor temperatures.
Keys to resilient MEP design include having discussions with the interdisciplinary team early to ensure space is allocated, knowing which systems need to function at all times, understanding the level of redundancy that is desired and that the project budget can support and recognizing how all of the systems work together. This is a team effort that continues as the design evolves.
Preparing for disaster
COVID-19 is spurring people to act. We are taking responsibility for social well-being through social distancing and staying home. We are supporting front-line medical professionals by 3D printing personal protective equipment components.
As engineers and building design professionals, we are tasked with the protection of the health, safety and welfare of the public. We have the power to do more. We can lead the way in helping our communities and institutions prepare for disaster before it strikes. We can design systems and buildings to withstand catastrophe and adapt to the next hazard event, from designing hospitals to convert more rooms into intensive care in a pandemic, to preparing for record-breaking storms.
By collaborating with architects, planners, civil engineers and landscape architects, we can collectively shape our built environment to be adaptive and resilient to the threats from Mother Nature. We can prepare not only for what we know to exist, but for anticipated changes in the future and the disruptions that we hope never to occur. The result: stronger communities, fewer lives lost and reduced risk of capital losses.
Now is the perfect time to talk about disaster preparedness and designing for resilience. Use the tools and strategies outlined here as a guide to leading clients and peers to adaptive, resilient design solutions.
Understanding a building’s resilient design levels
American Society of Civil Engineers 41 Resilience Levels is an engineering standard that can be used to determine the level of resilience critical to maintaining or returning to everyday operations. Resilience levels are defined in terms of potential harm to occupants, initial extent of damage and time and cost to regain functionality.
Life safety: Occupants inside are safe during a hazard event. The building loses functions and requires extensive repairs, leading to downtime while it is uninhabitable. After the event, occupants may be displaced for an extended period of time. This is the performance level embodied in the building code. Common examples: offices or residences.
Immediate occupancy: In addition to protecting any occupants during a hazard event, the facility is able to return to full functionality within 48 hours. Repairs are minor and repair materials and labor are readily available. This level of resilience is typically employed for facilities that serve an important function, but that can tolerate short-term interruptions in service. Common examples: outpatient health care facilities, redundant utilities and first responder support facilities.
Continuous operation: A facility designed for continuous operation during a hazard event has no loss of operations. This is an appropriate level of resilience for facilities whose loss of service would cause greater harm to the community. Common examples: major utilities, hospitals and data centers.
Additional resources:
- Climate Central
- Department of Housing and Urban Development Community Development Block Grant Disaster Recovery
- Environmental Protection Agency Drinking and Wastewater Resilience
- Federal Emergency Management Administration
- RELi Rating Guidelines for Design and Construction
- States at Risk
- S. Climate Resilience Toolkit